Mimicking Life: The Organ-on-Chip and the Future of Modern Science
Consider a scenario where the workings of a human heart, lung, or liver could be mimicked on a chip the size of a credit card. This might sound like science fiction, but it’s the exciting reality that Organ-on-Chip (OoC) technology is bringing to modern science. As researchers continue to explore the complexity of human biology, OoCs are emerging as powerful models to simulate the essential behaviors of human organs in the lab, driving breakthroughs in drug testing, disease modeling, and even personalized medicine. Let’s look at the story of this fascinating innovation and its game-changing impact on biomedical research.
A New Approach to Studying Human Organs
Suppose you were a scientist examining the effects of a drug on the heart. Typically, you might start with Petri dishes or animal models, neither of which fully reflect human physiology. Cells grown in a flat 2D environment don’t behave like those in a living body, and animals, while useful, are far from perfect models for human responses. This is where OoC technology comes in.
Organ-on-chip is a miniature system designed to mimic the physiomechanical behavior of human organs. In essence, it’s like taking the fundamental unit of an organ—say the tiny functional unit of a liver or a lung—and recreating its most essential features on a chip. These chips don’t aim to replicate an entire organ but rather recreate critical aspects such as fluid flow, mechanical forces, and biochemical signals that are vital for organ function. This means researchers can now work with a more realistic model of human tissues.
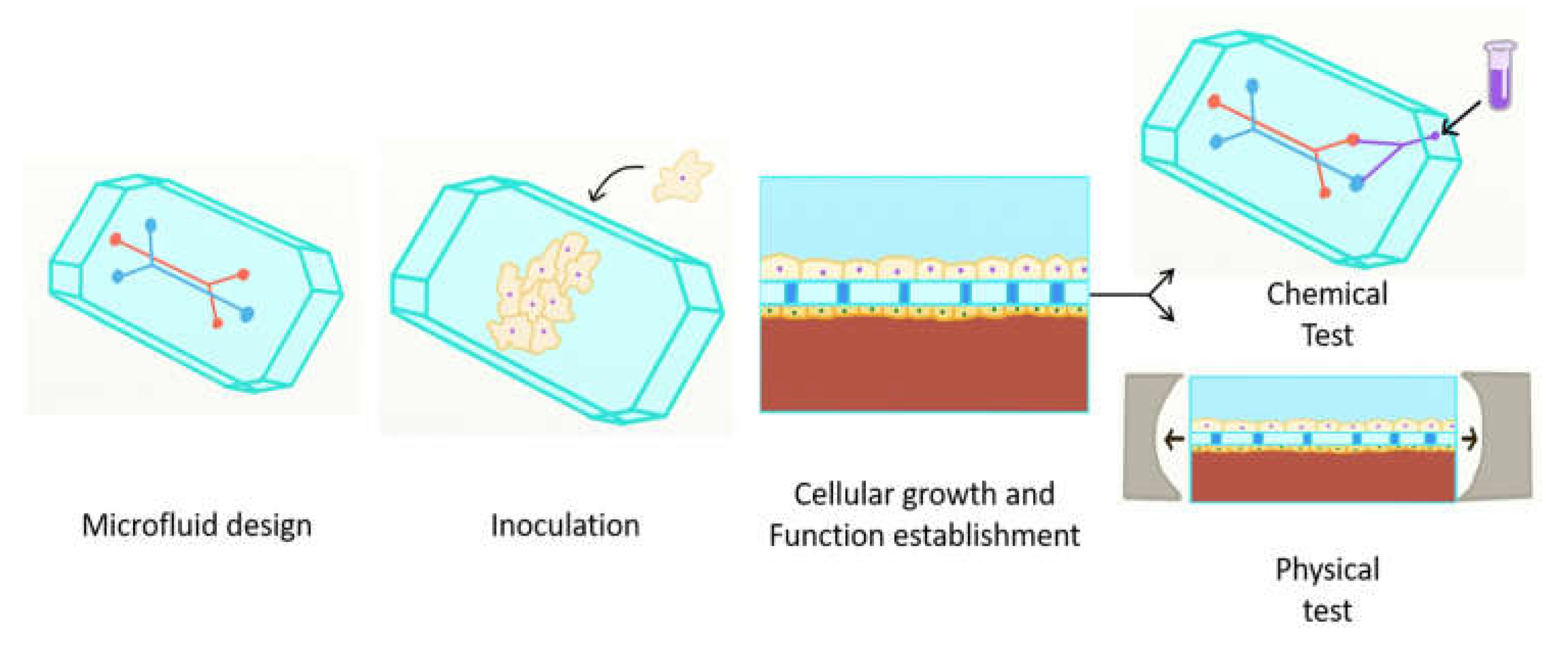
These chips, made from advanced materials and featuring micro-sized channels, simulate what happens inside the body: blood flowing through vessels, air passing through lung tissues, or even bile moving through the liver. It’s like having a tiny, transparent version of an organ you can manipulate and study under a microscope.
Improving Biomedical Research with Organ-on-Chip
What makes these chips so revolutionary? Organs inside the human body exist in highly dynamic environments. Cells don’t just sit passively; they experience fluid flow, compression, stretching, and other mechanical forces influencing their behavior. OoCs capture this complexity. For example, a chip for a lung can simulate the rhythmic expansion and contraction as a person breathes, while a chip mimicking the liver can show how it processes nutrients or toxins under real-time conditions.
Pharmaceutical companies have eagerly embraced OoCs. Testing new drugs on human-like systems in the lab not only reduces the reliance on animal models but also increases the chances of predicting how those drugs will behave in humans. In this way, OoCs help improve the accuracy of preclinical testing, making drug development faster, cheaper, and more ethical. The possibility of catching a potential drug failure before it enters human trials could save years of research and millions of dollars.
OoCs also hold enormous potential for personalized medicine. With advances in stem cell technology, creating chips from a patient’s cells is now possible. This means scientists can create disease models specific to an individual and test treatments tailored to that person’s unique biology. It’s like having your own custom-built mini organ in the lab, helping doctors make more informed decisions about your treatment.
What’s Happening Inside an Organ-on-Chip?
OoC systems carefully replicate the microenvironments found in human tissues. Let’s take a closer look at the key factors shaping their environment.
- Fluid Flow: Blood doesn’t just sit around in the body; it flows, delivering nutrients and removing waste. Similarly, in OoCs, fluid flow’s direction, speed, and pressure can be controlled to simulate nutrient delivery and waste removal, much like in natural tissues.
- Physical Forces: Besides fluid flow, cells are constantly subjected to forces like shear stress, compression, and stretching in our organs. OoCs recreate these mechanical environments, which is necessary for replicating how cells behave in living tissues. For instance, lung cells stretch when you breathe, and vascular cells experience compression and relaxation as blood pulses through your veins.
- 3D Cell Organization: Unlike traditional 2D cell cultures, OoCs allow cells to grow in three dimensions, closer to how they exist in real organs. This 3D architecture enhances cell-to-cell interactions, which are critical for proper tissue function and for studying diseases like cancer, where cell behavior and communication are significant factors.
- Substrate Stiffness: The material on which cells grow—the substrate—affects how they develop. In OoCs, materials can be fine-tuned to mimic the stiffness of different tissues, influencing everything from cell behavior to how they respond to treatments.
- Environmental Conditions: OoCs can replicate disease states by mimicking the inflammatory or pathological conditions that cause tissues to stiffen or become dysfunctional. This gives researchers a unique window into how diseases progress and how potential treatments could reverse these changes.
- Device design: The materials selected for the chip influence cell function, including adhesion, growth, and response to stimuli. Also, the microarchitecture of the device, including compartmentalization and confinement of cells, contributes to mimicking organ-level complexity.
The Promise of Organ-on-Chip Technology
OoCs aren’t just an incremental step forward in scientific research—they represent a fundamental change in approach. By accurately mimicking the human tissue’s mechanical properties, these miniature platforms are helping researchers better understand the complex dance of biology, disease, and treatment. From personalized medicine to drug discovery, the potential applications seem endless.
As OoC technology continues to evolve, the once-distant dream of creating a “human-on-chip” is becoming more realistic. With these tiny systems mimicking life, science and medicine’s future feels much closer than ever before.
Disclaimer
This blog simplifies complex scientific information for general understanding. The content is meant for educational purposes, highlighting advances in mechanobiology and disease investigation. Readers are encouraged to consult scientific articles for detailed insights and to recognize the broader context of this research within the scientific community.
References
- Leung, CM, et al. A guide to the organ-on-a-chip. Nat Rev Methods Primers 2020 May12; 2, 33. doi: 10.1038/s43586-022-00118-6.
- A guide to organs-on-chips (OoC) technology. White paper. Fluigent
- Thompson CL, et al. Mechanical Stimulation: A Crucial Element of Organ-on-Chip Models. Front Bioeng Biotechnol. 2020 Dec 10; 8:602646. doi: 10.3389/fbioe.2020.602646. Erratum in: Front Bioeng Biotechnol. 2021.
- Kutluk H, et al. Integration of Extracellular Matrices into Organ-on-Chip Systems. Adv Healthc Mater. 2023 Aug;12(20):e2203256. doi: 10.1002/adhm.202203256.